Last week, I was told my other brain is fully grown. It doesn’t look like much. A blob of pale flesh about the size of a small pea, it floats in a bath of blood-red nutrient. It would fit into the cranium of a foetus barely a month old.
Still, it’s a “brain” after a fashion and it’s made from me. From a piece of my arm, to be precise.
I’m not going to pretend this isn’t strange. But neither is it an exercise in gratuitously ghoulish biological engineering, a piece of Frankensteinian scientific hubris 200 years after Mary Shelley’s tale. The researchers who made my mini-brain are trying to understand how neurodegenerative diseases develop. With mini-brains grown from the tissues of people who have a genetic susceptibility to the early onset of conditions such as Alzheimer’s, they hope to unravel what goes awry in the mature adult brain.
It’s this link to studies of dementia that led me to the little room in the Dementia Research Centre of University College London last July, where neuroscientist Ross Paterson anaesthetised my upper arm and then sliced a small plug of flesh from it. This biopsy was going to be the seed for growing brain cells – neurons – that would organise themselves into mini-brains.
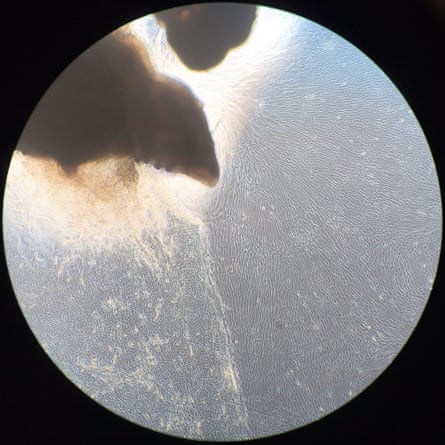
The Brains in a Dish project is one of many strands of Created Out of Mind, an initiative hosted at the Wellcome Collection in London and funded by the Wellcome Trust for two years to explore, challenge and shape perceptions and understanding of dementias through science and the creative arts. Neuroscientist Selina Wray at UCL is studying the genetics of Alzheimer’s and other neurodegenerative diseases and she and her PhD student Christopher Lovejoy gamely agreed to culture mini-brains from cells taken from four of the Created Out of Mind team: artist Charlie Murphy, who is leading Brains in a Dish, BBC journalist Fergus Walsh, neurologist Nick Fox and me.
It was a no-brainer… well, you know what I mean. Who could resist the narcissistic flattery of having another brain grown for them? I was curious how it would feel. Would I see this piece of disembodied tissue as truly mine? Would I feel protective of, even concerned for, a tiny “organoid” floating in a petri dish? Most of all, I was attracted by the extraordinary scientific feat of turning a lump of arm into something like a brain.
There’s a lot of baggage in that “something like”. Some researchers dislike the term “mini-brain” and with reason. This pea-size object is not a miniature version of the brain in my skull. It’s not even quite like the immature developing brain of an early-stage foetus. Without a body, neurons don’t quite know how to make a proper brain.
But neither are mini-brains blobs of identical neurons, like, say, a small chunk of my cortex. One can fairly say that the neurons “want” to make a brain but, lacking proper guidance, don’t quite know how to go about it. So they make a reasonable but imperfect approximation.
The mini-brain contains several types of brain cell, arranged somewhat as in a real brain – in layers such as those of the cortex, for example. The mini-brain even contains sketchy little versions of the folds and grooves on the surface of a true brain and appendages that, in a foetal brain, would become the brain stem and central nervous system, extending down the spine.
What’s most astonishing about this project is that these neurons started out as a piece of my arm. Those skin-forming cells, fibroblasts, were turned into brain cells using a technique discovered barely 10 years ago and that has revolutionised tissue engineering and embryo research and won its creator, Shinya Yamanaka, a Nobel prize. It also overturned decades of conventional wisdom in cell biology.
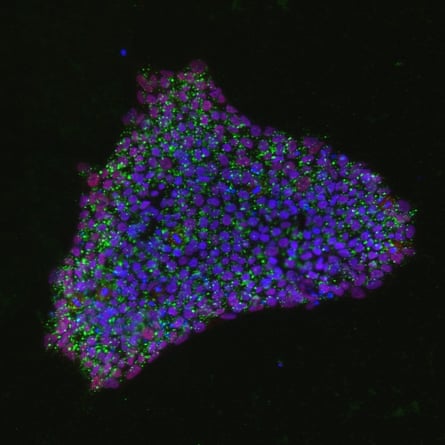
Our bodies grow from a single cell – a fertilised egg – by cell division accompanied by increasing cell specialisation. In the very earliest days of an embryo’s development, all its cells are capable of growing into any kind of tissue in the body. These are called embryonic stem cells and their complete versatility is called “pluripotency”.
As the embryo grows, some cells become committed to particular fates – they become skin cells, liver, heart, brain or bone-forming cells and so on. This differentiation springs from a modification of the cells’ genetic programme: the switching on and off of genes. As they differentiate, cells may change their shapes as well as their functions. Neurons grow the long, thin appendages that wire them into networks, the ends equipped with synapses where one cell sends an electrical signal to others. That signalling is the stuff of thought.
It was believed cell differentiation was one way – that once a cell was committed to a fate, there was no going back and that the silenced genes were switched off for ever. So it came as a surprise to many researchers when, in 2007, Yamanaka, a biologist at Kyoto University, reported that he could convert differentiated human cells directly back to a stem-cell-like state by adding to them the genetic material for making certain types of protein.
Yamanaka and his colleagues used viruses to inject into the mature cells some of the genes that are highly active in embryonic stem cells and they found that just four of these were enough to switch the cells into a pluripotent state, becoming, to all intents and purposes, like stem cells. These became known as induced pluripotent stem cells (iPSCs).
In principle, iPSCs can be grown outside the body into any tissue type, perhaps even into entire organs such as a pancreas or kidney, to replace a malfunctioning one by transplantation. Organs could be grown from cells – taken, like mine, from an arm, say – of the recipient, thus avoiding problems of immune rejection.
Creating organs involves knowing how to guide iPSCs towards the appropriate fate. This might involve giving them an extra dose of the genes that are highly active in that particular tissue type. But Chris turned my own iPSCs into neurons simply by changing the nutrient medium; such stem cells seem to have a preference for becoming neurons, so they only need a nudge to get them going.
Organs aren’t just big masses of a single cell type, however. The heart, kidney, brain and so on all contain many types of cell, organised in particular ways and fed with a blood supply. Reproducing that complex architecture in organs grown outside the body remains a huge challenge.
Yet cells can do a lot of it themselves. The biologist Madeline Lancaster discovered this when she was studying the growth of neurons from stem cells as a doctoral student in Vienna with the neuroscientist Jürgen Knoblich in 2010. She found that the neurons, left to their own devices, would start to specialise and organise into mini-brains.
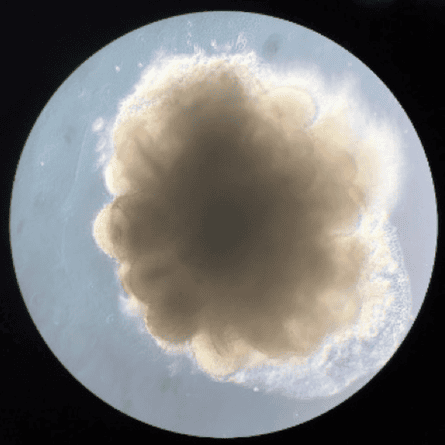
The plan, Lancaster (who now runs her own lab at the University of Cambridge) told me, was that she would make flat neural structures called rosettes, which had been done before. But the mouse stem cells she worked with wouldn’t stick well to the surface of the dishes. Instead, says Lancaster, “they formed these really beautiful 3D structures. It was a complete accident.”
Once they realised what they had made, she and Knoblich started to grow the structures from human stem cells, too. “At first, it was totally surprising that these cells could make a structure rather like a brain all by themselves”, she says. But in retrospect, she says, it makes complete sense. That kind of self-organisation is “just what an embryo does.” And it’s what I can now see in my own mini-brain, the different cell types stained with fluorescent dye to become a beautiful, multicoloured constellation under the microscope.
Lancaster and others are now seeking to find ways to supply mini-brains with more of the environmental cues they would get in a developing foetus, so that they can become even more brain-like. “You don’t need a completely well-formed human brain in a dish to study biological questions,” she explains. But if you can improve the resemblance in the right respects, you’ll get a better picture of the process in real bodies.
Lancaster uses brain organoids to investigate how the size of the human brain gets fixed. She has studied microcephaly, a growth defect that results in abnormally small brain size, and is also interested in what can make brains grow too big, which, contrary to what you might expect, is not a good thing and is linked to neurological disorders such as autism.
Other researchers are using these mini-brains to study conditions such as schizophrenia and epilepsy. At UCL, Wray is making them to understand the neurodegenerative process in two types of dementia: Alzheimer’s and frontotemporal dementia. The atrophy of brain tissue may start when two proteins called tau and amyloid beta switch from normal to misshapen form. These forms stick together in clumps and tangles that accumulate in the brain and cause neurons to die.
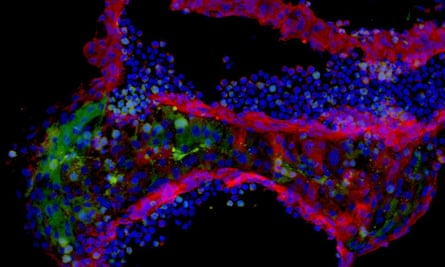
By culturing mini-brains from the cells of people with a genetic predisposition to these diseases (who account for about 1% to 5% of all cases), Wray hopes to find out what goes awry with the two proteins as neurons grow. “We are making mini-brains to try to follow the disease in real time,” she says. “We hope to see the very earliest disease-associated changes – that’s important when we think about developing treatment.” She has found that the tau proteins for the disease samples are different from those in healthy samples. My cultures may eventually be anonymised and used as one of those control samples.
How do I feel about these pieces of me growing in dishes in the centre of the city, six miles away from where I live? I was surprised to discover that they are no longer, officially, pieces of me at all. Cells that have divided outside the body are not classed as samples of tissue from an individual, but as “cell lines” – more nebulous entities that are distinct from their original donor.
Yet I do think of these brain organoids as “mine”, although not with any sense of ownership or pastoral duty. That’s probably a common response in people whose cells are cultured in the lab. The cancer cells taken in 1951 from the patient Henrietta Lacks at Johns Hopkins University hospital in Baltimore just before she died, and used for research (without her consent, which was not then required), are still regarded by Lacks’s surviving family as in some sense “her”, as Rebecca Skloot described in her bestselling 2011 book The Immortal Life of Henrietta Lacks. These “HeLa” cells are now the standard cell line for studying cancer and millions of tonnes of them have been grown worldwide: a piece of a person turned into a mass-produced commodity.
I’ll be very glad if my mini-brain can contribute in some small way to Wray’s research. I do not fear that it will have anything like anguished thoughts, any awareness at all, in its Matrix-like nutrient bath. But it does still seem like a piece of me, a wistful little attempt to remake the brain I take so much for granted. We have no frame of reference for thinking about such things. It is exciting and odd. But it’s also a glimpse of the future.
Comments (…)
Sign in or create your Guardian account to join the discussion